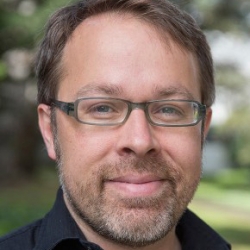
Scott Roy
( He/Him/His )Fields: Cell and Molecular Biology, Ecology and Evolution
At SF State Since: 2012
Specialties: Bioinformatics, Molecular Evolution
I am interested in the evolution of genome structures and the evolutionary origins of the pronounced differences in genome structure between different species. To study these questions, I study genomic sequences from a wide variety of species (and sometimes from different individuals within a species) to reconstruct evolution. I am interested in a wide variety of questions, and I encourage interested students to contact me to talk about possible projects.
Introns and Evolution:
My work focuses on the intron-exon structures of eukaryotic genes. Unlike prokaryotes (bacteria and archaea), most genes in the nucleus of most eukaryotic organisms are interrupted by a spliceosomal introns, thus gene expression requires splicing of RNA transcripts to remove these intervening sequences.
Spliceosomal introns are ubiquitous features of eukaryotic genomes. The average human transcript contains nearly ten introns, and intron removal requires a massive spliceosomal machinery comprising five catalytic RNAs and hundreds of proteins. Splicing is a major mode of proteome diversification in eukaryotes, and has key roles in gene regulation at the levels of transcription, transcript processing, nuclear export, and translation. Splicing has connections to chromatin structure, cancer, development, and a wide range of cellular processes, and alternative splicing affects 95% of multi-exon human genes. For such a multi-functional and integrated system, splicing shows remarkable diversity across species: eukaryotes show orders-of-magnitude differences in intron number (<10 to 200,000 per genome) and intron length (median from 19 to >2000 bases), and pronounced differences in splicing signals (from diffuse splice signals to strict extended motifs) and incidence and type of alternative splicing. These differences imply dramatic, recurrent, genome-wide changes in intron-exon structures throughout evolution, with myriad functional implications.
We work on several questions related to the evolution and function of introns and alternative splicing in eukaryotes.
The Big Questions:
- Why are there introns? At the most basic level, introns are surprising and unexpected. Bacteria (and archaea) get along fine without them, and many of them come and go in eukaryotic evolution, suggesting that many introns are dispensable. Moreover, even for those introns that do perform vital functions (most famously, alternative splicing to generate multiple products from the same gene – see below), the introns themselves generally appeared in evolution long before these functions (thus these functions cannot explain the origin of the intron themselves!) Thus there is a fundamental question as to why introns exist – in general, are introns beneficial or harmful (or just neutral) to an organism's fitness?
- Why do different species have such different intron-exon structures? While (nearly) all eukaryotic species have some spliceosomal introns, intron-exon structures vary strikingly across species, with huge differences in intron number, intron length, incidence and mode of alternative splicing, and intron sequences. These imply very different evolutionary histories of different species since the common ancestor of eukaryotes. What explains these qualitative differences in intron-exon structure across species?
- How does alternative splicing evolve? Alternative splicing involves differential splicing of portions of RNA transcripts from a single gene, leading to multiple different products. Alternative splicing performs a dizzying array of functions in animals and other eukaryotes, and understanding how these novel functions arise is a central question of comparative and evolutionary genomics. We are working on reconstructing the evolutionary history of alternative splicing in metazoans and reconstructing evolution in deep animal ancestors. In addition, we are interested in the evolution of the networks by which alternative splicing is regulated.
Ongoing Work:
We are working on a variety of projects attacking these various questions.
- Population genomics of intron loss and gain. Understanding the mechanisms and evolutionary forces that govern intron loss and gain requires identifying and studying recent changes in intron-exon structures. We are using comparative genomics to study very recent changes – those that are so recent that they differ between members of the same species. Studying these changes allows us to understand how intron-exon structures change, and how and why selection is acting on them.
- Diversity of intron-exon structures. We are studying intron-exon structures in a wide variety of eukaryotic lineages (mostly protists) to understand the diversity of intron-exon structures across species. Discoveries have included trans-spliced introns in the intestinal parasite Giardia lamblia, novel splicing mechanisms in the intracellular parasite Encephalitozoon cuniculi, and emergence of two novel classes of introns in another species (yet unpublished).
- Evolution of splicing networks. We are studying the evolution of splicing regulation in metazoans by reconstructing the evolutionary history of key regulatory networks. For example, we studied history of Nova, a family of proteins that regulates splicing of hundreds of genes in the vertebrate brain. We found that Nova is actually a very ancient splicing regulator, and that the brain-specific function of Nova is a relatively recent innovation. We are currently expanding this work to different species and different networks.